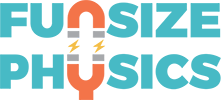
You may know that the media used in magnetic recording technologies, such as computer hard drives, are made of millions of tiny nanomagnets. Each nanomagnet can be switched up or down to record bits of information as ones and zeros. These media are constantly subjected to magnetic fields in order to write, read, and erase information. If you have ever placed a magnet too close to your laptop or cell phone, you know that exposure to an external magnetic field can disrupt information stored this way. Did you know that it is possible for the nanomagnets to "remember" their previous state, if carefully manipulated under specific magnetic field and temperature conditions? Using a kind of memory called topological magnetic memory, scientists have found out how to imprint memory into magnetic thin films by cooling the material under the right conditions.
When layered into thin films, magnetic materials known as ferromagnets exhibit nanoscale magnetic domains, which form maze-like patterns of long interlaced strips, as shown in frame (a) of Fig. 2. In some of these strips, the magnetization points up, perpendicular to the film, while in others, the magnetization points down. Within each strip, however, adjacent atoms are magnetized in the same direction. If the material is exposed to a strong magnetic field, these magnetic patterns are erased as all the magnetic domains flip in the direction of the applied field. When the field is removed, magnetic domains will reappear, but the pattern will be a totally different shape—that is, it will have a different topology. Multilayers of the elements cobalt and palladium exhibit this kind of ferromagnetic behavior.
In a different kind of magnetic behavior, known as antiferromagnetic, the magnetization of adjacent atoms points in opposite directions. When cooled below a certain temperature, called the blocking temperature, antiferromagnetic materials can be "frozen" into this pattern. Thin films made of the elements iridium and manganese exhibit antiferromagnetism.
It's when ferromagnetic and antiferromagnetic films are combined that things really get interesting. When a material is cooled below the blocking temperature, magnetic interactions called exchange couplings appear between the ferromagnetic and antiferromagnetic layers, allowing the template that was "frozen" in the underlying antiferromagnetic layer to influence the formation of magnetic domain patterns in the ferromagnetic layer. At low temperatures, the direction of magnetization in the ferromagnetic layer can still be flipped by the application of a magnetic field; when the field is removed, however, the domain pattern in the ferromagnetic layer tends to revert back to its original conformation, to match the template imprinted in the antiferromagnetic layer. By cooling the material, we can induce this kind of memory, called topological magnetic domain memory, restoring the original pattern in the ferromagnetic layer even after it has been exposed to an external magnetic field.
But how do we know all this? We measure magnetic memory in a material using intense x-rays of a particular wavelength, specific to the properties of that material. When the x-rays encounter the material, they are scattered, and the scattering pattern detected downstream is a unique fingerprint of the magnetic domain pattern in the material. An example of such a scattering pattern is visible in the frame (b) of Fig. 2. Many of these scattering patterns are collected at different points during the magnetization process to produce a correlation pattern, shown in frame (c).
We have found that the degree of memory achieved depends on the cooling conditions, as shown in Fig. 3. If the material is cooled in the absence of magnetic field or under a moderate magnetic field, up to 100% magnetic memory may be achieved, but if the material is cooled under a strong magnetic field, memory is decreased to below 40% and is limited to specific phases of the magnetization process.
Understanding how magnetic domains form, and how to control the patterns they form, is useful to improve the reliability of magnetic memory. These findings have potential applications in developing new nanotechnologies that rely on topological magnetic memory to store information.