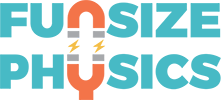
More data in less space—this has been the trend in electronics since the development of the first personal computer. Digital electronic devices like hard drives rely on magnetic memory to store data, encoding information as “0”s and “1”s that correspond to the direction of the magnetic moment, also known as spin, of the atoms in individual bits of material. The smaller these magnetic bits are, the more data can be stored in a given amount of material. For magnetic memory to work, however, it must also be stable—in other words, the magnetic moment of a material bit should not change until the data is accessed or rewritten. Magnetic moments in atoms tend to point in random directions, so it takes a lot of energy to align them in a preferred direction and keep them there over time. In the search for new nanomaterials that can host smaller bits for higher-density data storage, scientists are also looking for strategies to enhance the lifetime of the stored information in these materials, so the memory will not erase itself while you are still using the device!
One possible way to increase storage lifetime takes advantage of a material property called magnetic anisotropy, which locks the magnetic moment of a bit in a particular direction. The magnetic anisotropy energy of a material, represented by the red arrow in Figure 1, is the energy needed to change the direction of the magnetic moment. Materials with high magnetic anisotropy are good for stable data storage, since the spins will tend to stay aligned in one of two preferred directions, corresponding to a "0" or "1." Unfortunately, some materials that are otherwise well-suited to magnetic memory applications, such as Lanthanum Strontium Manganese Oxide [fusion_builder_container hundred_percent="yes" overflow="visible"][fusion_builder_row][fusion_builder_column type="1_1" background_position="left top" background_color="" border_size="" border_color="" border_style="solid" spacing="yes" background_image="" background_repeat="no-repeat" padding="" margin_top="0px" margin_bottom="0px" class="" id="" animation_type="" animation_speed="0.3" animation_direction="left" hide_on_mobile="no" center_content="no" min_height="none"][(La,Sr)MnO3 or LSMO], have low magnetic anisotropy. Researchers are working to find ways to increase the magnetic anisotropy of materials like LSMO, which will allow stable memory in novel magnetic memory devices.
One important factor affecting a material's magnetic anisotropy is its crystal structure. The preferred direction of magnetization generally depends on the arrangement of atoms in a crystal; when this arrangement is altered, the preferred magnetization will change. For example, spins in LSMO tend to align along the elongated axis or plane in the geometry of the crystal, referred to as the "easy axis" or "easy plane." Figure 2 shows how the horizontal x-axis and vertical y-axis are the same length in a cubic crystal, so the spins of atoms will be equally likely to align along the horizontal or vertical directions. By contrast, a distorted cubic crystal breaks the symmetry between the x- and y-axes, increasing the magnetic anisotropy of the material. When properly engineered, it is possible to enhance the magnetic anisotropy energy in the distorted cubic structure by more than 100 times compared with in the original cubic structure!
So how do we modify the crystal structure in a material like LSMO to increase its magnetic anisotropy and improve its usefulness for magnetic memory? One effective way is via strain, by squeezing (compressive strain) or stretching (tensile strain) the magnetic material. This can be achieved by clamping together materials that have similar crystal structure but different crystal sizes. In order to fit together, the crystal structures of these materials will be distorted. Figure 3 shows how the material LSMO will stretch to align its crystal structure with an adjacent material that has larger crystal size, creating a longer, lateral easy axis and locking in "left" or "right" spins, while it will squeeze to align with a material that has smaller crystal size, creating a longer, vertical easy axis and locking in "up" or "down" spins.
Through this approach, called epitaxial strain, we can modify a material's magnetic anisotropy, locking the magnetic moments in a preferred direction to create novel, stable magnetic memory devices.[/fusion_builder_column][/fusion_builder_row][/fusion_builder_container]