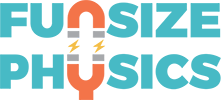
Imagine a magical material that sorts positive and negative charges to different positions, like rival teams on opposite sides of a volleyball court. This is exactly how ferroelectricity works! ~and the crowd goes wild~ Ferroelectric materials generate an electric field that moves charges around, just as a bar magnet produces a magnetic field that moves magnets around. And when we use a battery to switch the positive and negative charges (Fig. 1), ferroelectric materials can encode digital data—the familiar ones and zeroes of binary code—while using less energy than conventional magnetic memory.
The catch: ferroelectric materials don’t play well with the silicon technology used in devices like phones and computers. They’re like puzzle pieces that don’t fit together.
ENTER HAFNIA! ~triumphant kazoo band plays~ What the heck is hafnia, you ask? It's a molecule made up of one atom of hafnium (a metallic element) and two atoms of oxygen. Hafnia-based materials are already part of modern electronic devices, so a ferroelectric form of hafnia would fit the puzzle, play well with silicon, AND bring all the advantages of ferroelectrics. But hafnia behaves differently depending on how its atoms are arranged—only one arrangement, shown in Fig. 2, exhibits ferroelectric properties. (To learn more about how different atomic arrangements affect material properties, check out "The Shape of the Future"!) .
Grains on the Brain
Particle size also plays an important role in determining the properties of materials. In a stained-glass window, for example, many of the colors you see are not produced by different substances, but by different particle sizes of the same substance. In the case of hafnia and other crystals, we talk about grain size rather than particle size. Most crystals don't have uniform structure but are comprised of individual grains with edges. If you've ever eaten rock candy (Fig. 3), you know about crystal grains; while the grains in rock candy are large and easy to see with the naked eye, however, other crystals may look uniform but still contain distinct grains at the microscopic level (Fig.4). (Learn more about crystal grains in "Crystals and Spintronics"!)
Scientists used to believe ferroelectricity was only possible with small grains of hafnia, which seemed to lock the ferroelectric crystal arrangement in place. Unfortunately, small grains are also harder to study. But guess what? Recent research shows that hafnia was misunderstood all along!
Big Grains, Big Surprises
Using a technique called epitaxy, scientists grew a remarkable new type of hafnia crystal with grains hundreds of times bigger than the more familiar form of ferroelectric hafnia. Imagine building a tower of blocks: you start with a big base block, then you carefully add smaller blocks on top of it. Epitaxy works the same way, as we grow new crystal layers on top of an existing layer (Fig. 5).
These high-quality, large-grain crystals are easier to study, unlocking a treasure trove of new knowledge about ferroelectricity in hafnia. To scientists' surprise, they found big grains make hafnia even more ferroelectric than small ones! Better still, the ferroelectricity in large-grain hafnia crystals stays strong when cooled—even in freezing temperatures, when its smaller-grained cousins get a bit shaky, the large-grained hafnia retains its superpowers.
Next time you see a touchscreen or a memory chip, remember these superhero hafnia crystals and other ferroelectric materials might just be working their magic behind the scenes!